Research and innovation in physics and engineering
Cutting-edge interdisciplinary research and innovation to deliver real-world impact
We are at the forefront of pioneering research and technological development, seeking to make advances in physics and engineering that will shape the technologies of the future.
Our interdisciplinary approach links theory, practice and creativity as we explore fundamental phenomena and translate our expertise to high impact areas – from next-generation fusion reactors and autonomous robots to quantum technologies and healthcare.
As well as attracting significant funding from research councils, we have strong industry links, forming spin-out companies to bring innovative products to market.
Our discovery research themes
The strength and breadth of our fundamental research is reflected in eight core themes that champion originality, rigour, and excellence.
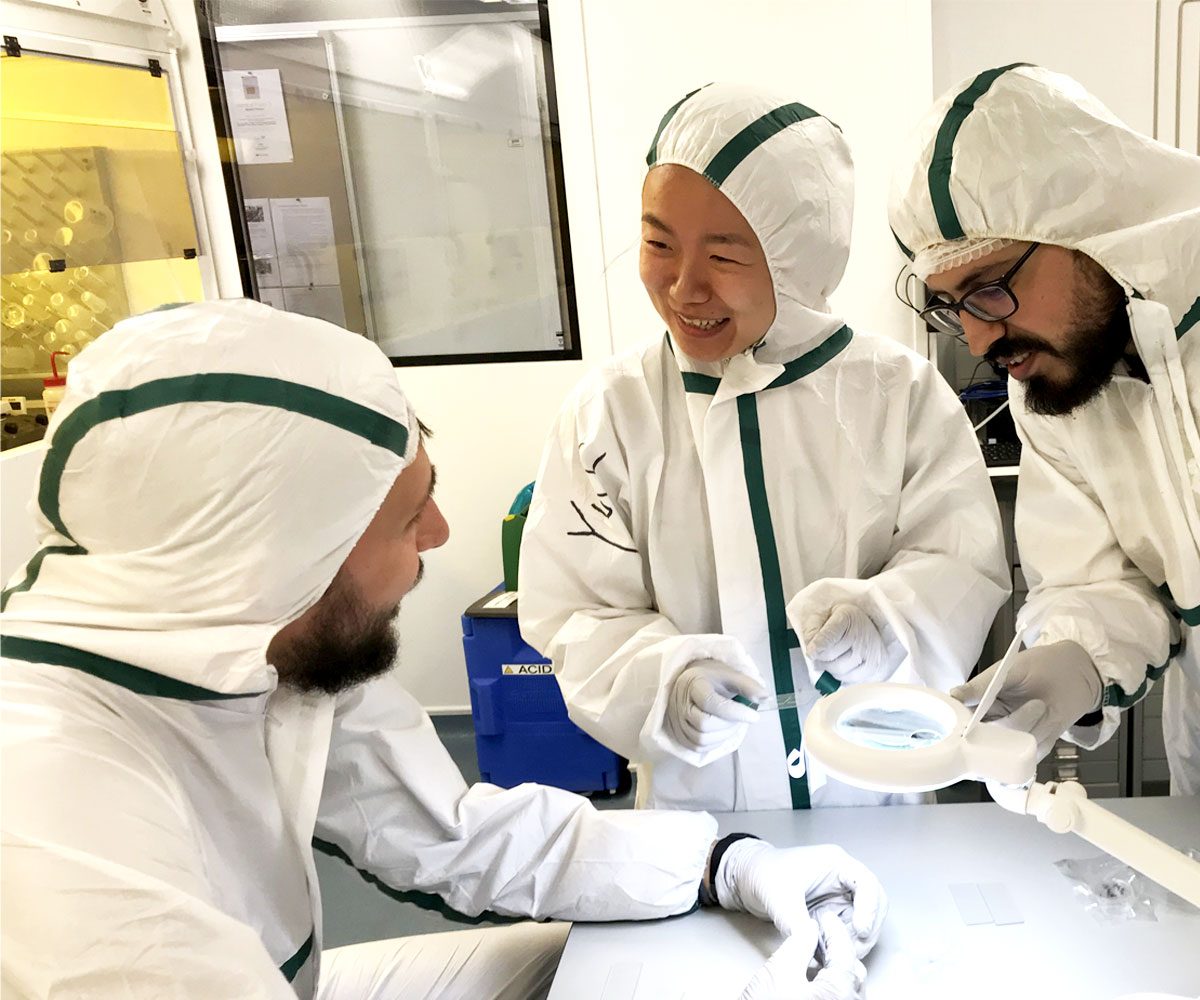
Driving innovation through translational research
Our translational research allows us to focus on real-world problems and train the next generation of innovators, often in collaboration with business partners and external organisations.
Our impact
Business and industry engagement
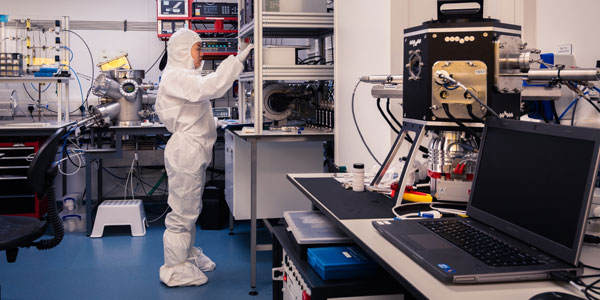
We’re keen to ensure that industries have access to all the school can offer, whether it’s finding the best graduate engineers and physicists, developing a product, using our facilities, or carrying out collaborative research.
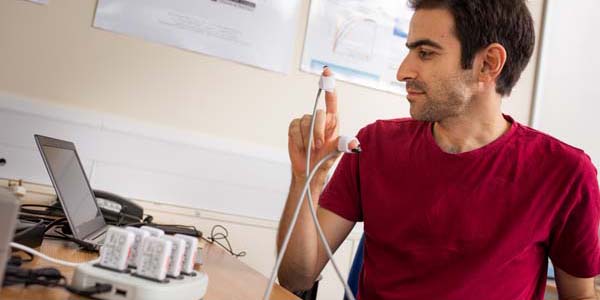
ClearSky Medical Diagnostics specialises in medical devices for the diagnosis and monitoring of Parkinson’s, Alzheimer’s and a range of other neurodegenerative conditions.
Commercialisation, consultancy and spin-out companies
Our academics undertake a wide range of commercialisation and consultancy activities, which have led to the following spin-out companies:
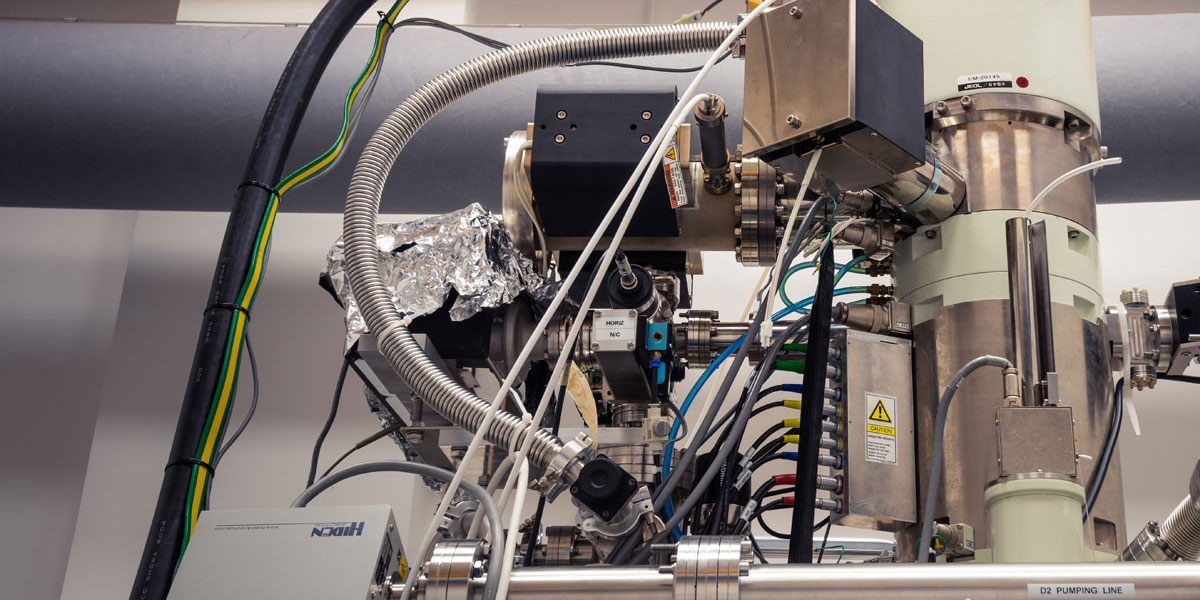
Centres and facilities
We drive progress in science, industry and policy through our cross-disciplinary research centres and state-of-the-art facilities.