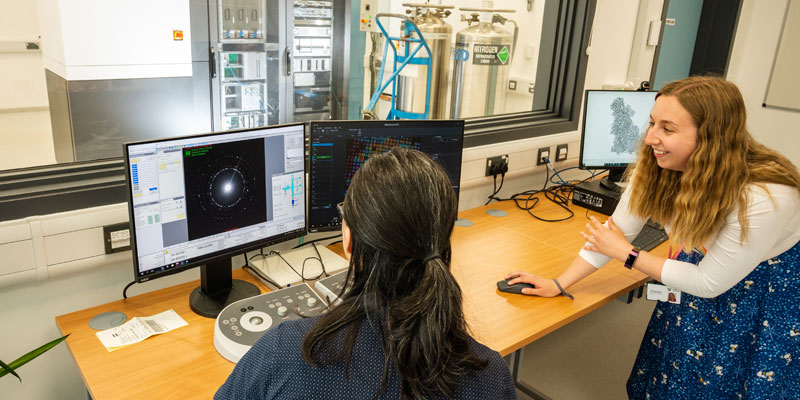
Electron cryo-microscopy
Electron cryo-microscopy (cryo-EM) has recently emerged as a powerful structural biology technique. York is well equipped with excellent facilities for cryo-EM, housed in the Eleanor and Guy Dodson Building.
Cryo-EM allows the user to directly image biological molecules and compute their three-dimensional structure. This approach can provide information on the structure of biological molecules and is well suited to exploring the dynamics of biological action on the molecular scale. Increasingly, cryo-EM can also be used to explore the structure of biological molecules within cells.
Techniques
Single-particle analysis is the most used cryo-EM technique. Here, the target is frozen in the holes of a cryo-grid using liquid ethane in a liquid nitrogen bath. As the sample is frozen so quickly, ice-crystals are avoided and the water enters the ‘vitreous ice’ state. Here, the ice is solid but disordered.
Once the grid is inserted into the microscope, many thousands of particles are imaged and a 3D structure is calculated by combining many different views of the particle. The resulting map of the particle can then be used in a very similar manner to a crystallographic map. Typically resolutions are regularly in the 3-4 Å region, although the number of maps between 2 and 3 Å is increasing, and there are now several maps of important targets below 2 Å.
The sample requirements for single particle analysis are very low compared to crystal trials. A minimum starting point would normally be about 20 μL of your purified target at about 1 mg ml-1, although different projects can require very different concentrations of protein.
Tomography is a rapidly growing image reconstruction technique, which is powerful for imaging cellular structures. Single-particle analysis relies on imaging many homogeneous particles in different poses whereas in tomography, a heterogeneous target is instead placed in many poses by tilting the stage and imaging the sample at each angle. Like single particle analysis, a map (or tomogram), is reconstructed computationally from each image.
The resolution of tomograms is limited, however, the homogenous elements of the structure within the tomogram can be averaged together to improve the signal-to-noise ratio and resolution.
Electrons can be used in just the same way as X-rays to determine the structure of crystals. As electrons interact with matter far more strongly than X-rays, very small crystals can be used, typically of the order of a few nanometres. For biological systems, there is much to learn by comparing electron and X-ray diffraction and for non-biological applications, the small size of the required crystals allows structures to be solved from drastically smaller quantities of material. For non-biological systems, microED allows the determination of the structure with minuscule quantities of material.
Scanning transmission electron microscopy (STEM) is an alternative imaging modality. Here, rather than illuminating the entire image with an electron beam, a small beam is focused onto a point on the sample and rastered across the sample. At each point, the elastically and inelastically scattered electrons are collected, and an image built up. In materials science, STEM is used to create very high resolution images and to simplify interpretation. For biological applications, STEM allows one to work with thick samples and to generate element-based contrast in maps. It can be used as the imaging modality in both single-particle analysis and tomography workflows.
The microscope
We have a 200 kV Thermo Scientific Glacios electron cryo-microscope, housed in a low-humidity, low-vibration, constant temperature space to ensure optimal performance with minimal ice contamination. While primarily used for biological ‘single particle analysis’, the York Glacios is designed to be a work-horse capable of a range of different imaging modes.
It is equipped with the following components to achieve this goal:
- A Falcon-IV counting direct electron detector. This highly sensitive detector allows us to measure high-resolution information in our images before the electron beam damages the sample
- A ceta-D for diffraction work. This is a robust detector that has a large dynamic range, suitable for electron diffraction studies
- Panther segmented STEM detectors. The segmented detectors have a total of four rings, of which each is further divided into four quadrants, providing 16 overall
- A Shottky field emission gun (FEG), which provides a coherent beam of electrons with a low energy spread suitable for high-resolution work
- An autoloader, which handles the delicate cryo-grids carrying our samples, keeping them constantly at close to liquid nitrogen temperatures and moving them into and out of the microscope with minimal contamination
- An expanded C2 aperture strip, which allows us to illuminate a small area of the grid whilst still maintaining parallel beam conditions, improving through-put and data collection rates.
Sample preparation
We have two Thermo Scientific Vitrobots for plunge-freezing grids. Like the microscope, the vitrobot is housed in low-humidity space, making it easy for even inexperienced users to prepare grids with minimal ice contamination.
Training
We have an active training program. For data collection we provide training ‘on-the-job’, i.e. the user learns sample preparation and microscope operation using their own material.
Computing
Data obtained on the Glacios are first stored on an offload server with 40 TB of SSD space. This server is connected using fibre optic to the cameras, as well as to our dedicated file server for cryo-EM data, currently with 480 TB of disk space, which will shortly be extended to 1.2 PB. Longer-term archiving is envisaged to be on Amazon Glacier. Data processing is carried out on a number of GPU compute boxes, and using the compute power provided by the HPC clusters (GPU and CPU) of the University, such as Viking.
Our publications
- Helena-Bueno, K., Rybak, M. Y., Ekemezie, C. L., Sullivan, R., Brown, C. R., Dingwall, C., Basle, A., Schneider, C., Connolly, J. P. R., Blaza, J. N., Csorgo, B., Moynihan, P. J., Gagnon, M. G., Hill, C. H. & Melnikov, S. V. A new family of bacterial ribosome hibernation factors. Nature 2024 626:1125-1132
- Woodgate, J., Mosaei, H., Brazda, P., Stevenson-Jones, F. & Zenkin, N. Translation selectively destroys non-functional transcription complexes. Nature 2024 626:891-896
- Viola CM, Frittmann O, Jenkins HT, Shafi T, De Meyts P, Brzozowski AM. Structural conservation of insulin/IGF signalling axis at the insulin receptors level in Drosophila and humans. Nat Commun. 2023 14(1):6271
- Goodall JC, Sajjad MA, Thompson EA, Page SJ, Kerrigan AM, Jenkins HT, Lynam JM, Macgregor SA, Weller AS. In crystallo lattice adaptivity triggered by solid-gas reactions of cationic group 7 pincer complexes. Chem Commun (Camb). 2023 59(72):10749-10752
- Bayfield OW, Shkoporov AN, Yutin N, Khokhlova EV, Smith JLR, Hawkins DEDP, Koonin EV, Hill C, Antson AA. Structural atlas of a human gut crassvirus. Nature. 2023 617(7960):409-416
- Hawkins DEDP, Bayfield OW, Fung HKH, Grba DN, Huet A, Conway JF, Antson AA. Insights into a viral motor: the structure of the HK97 packaging termination assembly. Nucleic Acids Res. 2023 51(13):7025-7035
- Bárdy P, MacDonald CIW, Pantůček R, Antson AA, Fogg PCM. Jorvik: A membrane-containing phage that will likely found a new family within Vinavirales. iScience. 2023 26(11):108104
- Silale A, Zhu Y, Witwinowski J, Smith RE, Newman KE, Bhamidimarri SP, Baslé A, Khalid S, Beloin C, Gribaldo S, van den Berg B. Dual function of OmpM as outer membrane tether and nutrient uptake channel in diderm Firmicutes. Nat Commun. 2023 14(1):7152
- Rowland RJ, Heath R, Maskell D, Thompson RF, Ranson NA, Blaza JN, Endicott JA, Noble MEM, Salamina M. Cryo-EM structure of SKP1-SKP2-CKS1 in complex with CDK2-cyclin A-p27KIP1. Sci Rep. 2023 13(1):10718
- Doyle LR, Thompson EA, Burnage AL, Whitwood AC, Jenkins HT, Macgregor SA, Weller AS. MicroED characterization of a robust cationic σ-alkane complex stabilized by the [B(3,5-(SF5)2C6H3)4]-anion, via on-grid solid/gas single-crystal to single-crystal reactivity. Dalton Trans. 2022 51(9):3661-3665
- Snow AJD, Sharma M, Abayakoon P, Williams SJ, Blaza JN, Davies GJ. Structure and mechanism of sulfofructose transaldolase, a key enzyme in sulfoquinovose metabolism. Structure. 2023 31(3):244-252.e4
- Li J, Sharma M, Meek R, Alhifthi A, Armstrong Z, Soler NM, Lee M, Goddard-Borger ED, Blaza JN, Davies GJ, Williams SJ. Molecular basis of sulfolactate synthesis by sulfolactaldehyde dehydrogenase from Rhizobium leguminosarum. Chem Sci. 2023 14(41):11429-11440
- Armstrong Z, Meek RW, Wu L, Blaza JN, Davies GJ. Cryo-EM structures of human fucosidase FucA1 reveal insight into substrate recognition and catalysis. Structure. 2022 30(10):1443-1451.e5
- Furlan C, Chongdar N, Gupta P, Lubitz W, Ogata H, Blaza JN, Birrell JA. Structural insight on the mechanism of an electron-bifurcating [FeFe] hydrogenase. Elife. 2022 11:e79361