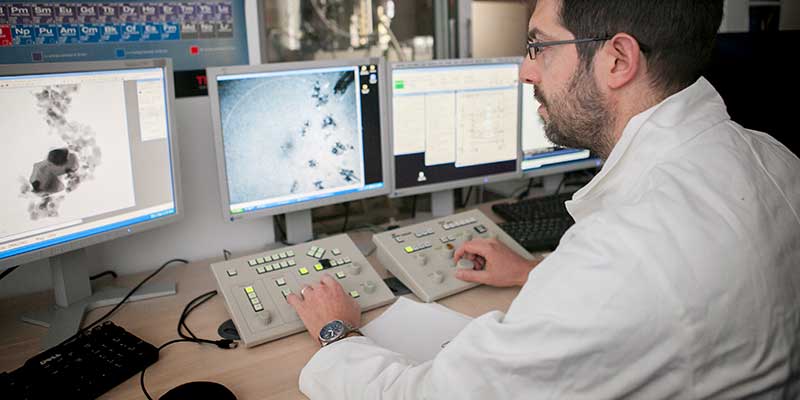
SusMat0 research projects and industry partnerships
We have four fully funded projects available for September 2024 start (providing three and a half years stipend at the UKRI recommended rate and fees). We also have projects available to applicants with their own funding (for example, through a scholarship). There are a wide range of scholarships and studentships available to international (including EU) and UK students.
- Discovery of new sustainable permanent magnets for electric motors and generators.
- Photonics for Net Zero Enabled by Patternable Boron-Phosphide Polymers.
- Towards Circular Solar: Advanced Methods for Recycling Solar PV Materials.
- Organic Synthesis and Self-Assembly of State-Independent Electrolyte Materials.
Entry requirements
You should have, or expect to obtain, the equivalent to a UK integrated Masters degree at 2:1 or above, or an MSc/MRes in Physics, Chemistry, Engineering or a related discipline.
We will also consider applicants from three year undergraduate degree programmes (for example, a UK BSc) with an equivalent of 2:1 or higher in a relevant discipline where additional industrial experience can be demonstrated. If you're a non-native English speaking applicant you must provide evidence of your English language ability.
How to apply
You can find details of available projects below. When you find the project or projects you would like to apply for, follow the link to start your application.
On the postgraduate application form, please select 'CDT in Sustainable Materials for Net Zero' as your source of funding. You do not need to provide a research proposal, just enter the name of the project you wish to apply for.
If you are interested in more than one project please make only one application and list all project titles.
We will continue to accept applications for projects open for applicants with external funding which will be assessed as they are received.
Funded projects
Prof Keith McKenna, Dr Stuart Cavill and Dr Xing Zhao
High-performance permanent magnets are needed for electric motors and generators which are essential for the decarbonisation of transport (land, sea and air) and energy generation (>25% of UK electricity comes from wind-turbines). Discovered in the 1980s, alloys of rare-earth metals (e.g., Nd–Fe–B and Sm–Co) remain the best performing materials with high saturation magnetization (Ms), Curie temperature (Tc) and magnetic anisotropy (Ku). However, the scarcity and environmentally-restricted nature of rare-earth metals, coupled with difficulties in recycling and rapidly growing global demand (~18% pa) means we are facing a significant sustainability challenge.
The aim of this project is to identify and develop urgently-needed sustainable alternatives to rare-earth magnets which combine high-performance with earth-abundance. For example, Fe–N based materials have recently emerged as a promising contender but challenges over stability, manufacturability and optimisation of composition remain.
In this project, you will deploy density functional theory (DFT) and DFT-trained machine-learning (ML) potentials to exploring a wider earth-abundant materials design space and screen prospective materials for desirable magnetic properties. Strategies to boost their performance (such as doping) and nanostructuring will also be investigated. High-quality thin-films of promising materials will be prepared by pulsed-laser deposition (PLD) and characterised structurally and magnetically. Through iterative feedback approaches to optimise material properties will be investigated and multi-physics simulations will be used to model prospective performance in devices.
The studentship is offered by the Centre for Doctoral Training in Sustainable Materials for Net Zero (SusMat0). SusMat0 is focused on the development of sustainable materials for advanced energy-related technologies key to achieving the target of net zero carbon emissions. It includes research on materials for energy generation/storage technologies (for example solar cells, batteries), devices with improved energy efficiency (for example OLEDs, memories, power electronics) and technologies for synthesising chemicals using renewable energy. As a member of a cohort of students, you will receive training in core chemistry, physics and engineering approaches relevant to cross-disciplinary sustainable materials research. We aim to produce well-rounded scientists, equipped and empowered to engage effectively with each other.
If you have any enquiries, please contact Professor Keith McKenna (keith.mckenna@york.ac.uk)
Professor Andrew Weller and Dr Christina Yue Wang
The manipulation of light in optoelectronic devices is a cornerstone for the delivery of Net Zero, for example in low-energy optical computing (silicon-photonics) and green hydrogen generation from water (photocatalysis). Underpinning such technologies are 2D/thin-film group III/IV/V semiconductors that have controllable bandgaps. Unlike graphene (zero bandgap) or hexagonal-boron-nitride (h-BN, 6 eV), boron-phosphide (BP) is a semiconductor (2 eV bandgap for cubic-BP, 1 eV direct bandgap predicted for h-BP), that also has additional attractive thermal (conducting), mechanical (super-hard) and chemical (oxidation-resistant) properties. However, c-BP is notoriously difficult to synthesise, while the synthesis of h-BP is unknown. Unlocking the reliable, scalable, and efficient synthesis of c-BP and h-BP will set the scene for their wider development and represent a breakthrough in main-group materials chemistry and III–V semiconductor utilisation for Net-Zero applications. In particular, c-BP is acknowledged as being a next-generation photocatalyst for water splitting (i.e. green hydrogen); while h-BP is predicted to be a perfect candidate for deployment in silicon emitters, the “Holy Grail” of optical computing.
This project will explore the synthesis and development of main-group phosphine-borane-polymers (polyphosphinoboranes) as scalable pre-ceramic precursors to c-BP or h-BP. These polymers will then be integrated with photonic devices, such as solar absorbers and silicon emitters, using thin-film methods and nanolithography techniques. The project will combine the expertise of Andrew Weller in the catalytic synthesis and exploitation of pre-cursor BP-polyphosphinoboranes [1] with expertise in photonic devices of Christina Wang [2].
The project will be suitable for a PhD candidate who has interests in innovative molecular synthesis methods for new, polymeric, pre-ceramic BP-polymers, and their deployment in technologically important photonic devices. The project will be truly interdisciplinary, crossing traditional boundaries. The PhD student would be based in both the Weller and Wang labs, with access to the state-of-the-art facilities including the nanofabrication facility at York JEOL Nanocentre, and will work with teams of experts in both groups to deliver on the ambitious goals. While full training will be given in all aspects of the project, it would best suit a graduate student with experience of synthetic chemistry who has a real interest in transitioning and expanding their skill set to include materials chemistry and device fabrication. There are opportunities for wider collaboration on the project (and international travel) to other centres of excellence in main group polymer synthesis and materials characterisation.
The studentship is offered by the Centre for Doctoral Training in Sustainable Materials for Net Zero (SusMat0). SusMat0 is focused on the development of sustainable materials for advanced energy-related technologies key to achieving the target of net zero carbon emissions. It includes research on materials for energy generation/storage technologies (for example solar cells, batteries), devices with improved energy efficiency (for example OLEDs, memories, power electronics) and technologies for synthesising chemicals using renewable energy. As a member of a cohort of students you will receive training in core chemistry, physics and engineering approaches relevant to cross-disciplinary sustainable materials research. We aim to produce well-rounded scientists, equipped and empowered to engage effectively with each other.
[1] J. Am. Chem. Soc. 2021, 143, 21010; Angew. Chem. Int. Ed. 2023, e202216106
[2] ACS Nano 2022, 16, 6493; Laser Photonic Rev. 2018, 12, 1800015.
If you have any enquiries, please email Professor Andrew Weller.
Mahmoud Dhimish (Primary Supervisor), Vlado Lazarov (Secondary Supervisor)
Project Description:
An exciting PhD opportunity is open at the University of York, Centre for Doctoral Training (CDT) in Sustainable Materials for Net Zero (SuMat0), where the appointed researcher will be instrumental in forging new pathways for recycling solar photovoltaic (PV) materials. The project will tackle the complex challenge of sustainably managing end-of-life PV panels through the development of advanced recycling processes that bridge the gap between academia and industry standards.
Research Methodology:
The methodology adopted for this PhD project is a comprehensive approach that synthesises chemical and thermal treatment strategies to recover and repurpose materials from PV modules. The student will design and execute a series of experiments beginning with:
Chemical Leaching Techniques: The researcher will implement an innovative two-stage leaching process. The primary stage will focus on the solubilisation of aluminium using a carefully prepared solution, optimising the concentration, temperature, and time to maximise yield. The secondary stage will be dedicated to the recovery of silver, employing an organic solvent mixture to dissolve and subsequently precipitate silver chloride, a valuable material for reclamation.
Optimised Thermal Treatments: In parallel, the candidate will develop thermal treatment protocols to disassemble the PV modules and separate the encapsulant from the glass and silicon layers. They will explore different temperature profiles and atmospheres to determine the most effective conditions for material separation that prevent degradation and facilitate the reuse of silicon and glass in new modules.
Material Characterisation and Analysis: Post-treatment, the candidate will utilise a battery of analytical techniques. Advanced microscopy methods like TEM and SEM will be used to assess the morphology and composition of reclaimed materials. AFM will provide nanoscale surface characterisation, vital for understanding the structural integrity of recycled silicon wafers. Additionally, the project will incorporate EL/PL imaging techniques to assess the optoelectronic quality of the silicon material, essential for determining the efficiency potential of recycled PV cells.
Throughout this experimental phase, the student will be expected to fine-tune process parameters to improve the efficacy and environmental footprint of the recycling process. A life cycle assessment will be conducted to ensure the new methodologies not only meet the standards for material recovery but also contribute positively to the overall sustainability of the PV industry. It is the goal of this approach to achieve >95% glass reuse, >90% metal recovery, and up to 85% silicon reuse. As a result, nearly 85% of the PV materials can be extracted efficiently in this way, which is in alignment with the current EU/UK WEEE regulations.
Project Impact:
The expected outcome of this research would be the creation of a scalable, economically feasible, and environmentally friendly PV recycling protocol. Not only will this alleviate the growing waste management problem, but it will also create a blueprint for future research and development regarding the treatment of PV end-of-life materials in a sustainable way.
Application Process:
Candidates with a background in engineering, physics, materials science, chemistry, or a related field with industrial experience are encouraged to apply. Please submit your CV, a cover letter detailing your research interests and experience, and at least two academic or professional references.
Join us in developing the next generation of sustainable PV recycling technologies and make a lasting impact on the future of renewable energy!
Centre for Doctoral Training in Sustainable Materials for Net Zero (SusMat0)
The studentship is offered by the Centre for Doctoral Training in Sustainable Materials for Net Zero (SusMat0). SusMat0 is focused on the development of sustainable materials for advanced energy-related technologies key to achieving the target of net zero carbon emissions. It includes research on materials for energy generation/storage technologies (for example solar cells, batteries), devices with improved energy efficiency (for example OLEDs, memories, power electronics) and technologies for synthesising chemicals using renewable energy. As a member of a cohort of students you will receive training in core chemistry, physics and engineering approaches relevant to cross-disciplinary sustainable materials research. We aim to produce well-rounded scientists, equipped and empowered to engage effectively with each other.
For any informal inquiries about this project, please contact Dr. Mahmoud Dhimish, the primary supervisor, at Mahmoud.Dhimish@york.ac.uk
Ideal electrolytes for energy devices such as batteries and solar cells would (i) be lightweight and flexible, (ii) exhibit thermal stability, (iii) be non-volatile (to reduce fire risk), (iv) provide good interfacial contact with electrodes, (v) be amenable to processing and recycling, (vi) have high ionic conductivities, and (vii) be made from cheap, renewable feedstocks. There are currently no electrolytes (inorganic solids, organic liquids, or composites) that meet all these criteria simultaneously.
This PhD project aims to develop a series of electrolytes that meet these criteria. In particular, we aim to use organic and supramolecular chemistry concepts to design electrolytes that retain liquid-like ionic conductivity in the solid state, i.e., they act as state-independent electrolytes. The project is suited to students who have interests in organic materials chemistry and synthesis, and who are excited by working in an interdisciplinary team.
The student will be trained to carry out the organic synthesis of state-independent electrolytes and to characterise their conductivity and other materials properties under the supervision of Dr McGonigal in the Department of Chemistry. Their experiments will be guided by simulations of the ionic conduction, which the student will learn to perform under the guidance of Prof Probert in the School of Physics, Engineering and Technology.
The studentship is offered by the Centre for Doctoral Training in Sustainable Materials for Net Zero (SusMat0). SusMat0 is focused on the development of sustainable materials for advanced energy-related technologies key to achieving the target of net zero carbon emissions. It includes research on materials for energy generation/storage technologies (for example solar cells, batteries), devices with improved energy efficiency (for example OLEDs, memories, power electronics) and technologies for synthesising chemicals using renewable energy. As a member of a cohort of students you will receive training in core chemistry, physics and engineering approaches relevant to cross-disciplinary sustainable materials research. We aim to produce well-rounded scientists, equipped and empowered to engage effectively with each other.
Projects open for applicants with external funding
Department of Chemistry
Dr Richard Douthwaite, Professor Vlado Lazarov
As wind and solar energy continue to make an increasing contribution to our electricity supply there is a growing need for effective storage to balance demand. Renewable energy storage in a chemical fuel is one option that could also make a significant contribution to some transportation needs particularly if coupled with CO2 capture and utilization. However, catalysts are required for chemical fuels synthesis using intermittent energy, which can operate with repeated cycling without degradation. This contrasts with the continuous steady-state processes typically used in industrial chemicals production. Recent developments in aberration-corrected environmental transmission electron microscopy (AC-eTEM) allow dynamic in-operando investigation of catalytic reactions with atomic precision.
We aim to use state-of-the-art AC-eTEM for dynamic in operando studies of catalysts used for CO2 to fuels. Specifically, iron catalysts are used for the hydrogenation of CO2 giving carbon coupled products. However, catalyst lifetime and product selectivity are key challenges, resulting from catalyst evolution due to structural and compositional changes, particle sintering, and coking. We aim to gain an atomic understanding of catalyst evolution in operando as a function of reactive gas atmospheres and temperature to help target catalysts resistant to repeated cycling and improve selectivity for carbon coupled products from waste CO2. A student will gain knowledge of the hydrogen economy, CO2 mitigation strategies and expertise in catalysis and advanced electron microscopy methods. As part of a cohort of students under SusMat0 further broader experience will be gained across materials synthesis, characterisation and modelling applied to meeting Net Zero targets.
If you have any enquiries, please email Dr Richard Douthwaite
Department of Chemistry
Professor Victor Chechik, Dr Mohammad Nasr Esfahani, Dr Richard Douthwaite
Light and electricity are the most sustainable and efficient energy sources for chemical reactions, and recent years saw an explosion of interest in photo- and electrocatalytic synthetic methodologies. Heterogeneous photocatalysts (eg, inorganic semiconductors) are particularly attractive as they offer simple product separation. However photochemical transformations with semiconductor catalysts often suffer from low efficiency, eg, due to competing electron-hole recombination. Coupling photo and electrocatalysis makes it possible to overcome this issue and significantly improve reaction efficiency.
The efficiency of photo and electrochemical processes can be significantly increased in continuous flow systems, as this decreases the distance between the electrodes and hence resistance losses, reduces light path length and increases surface to volume ratio. While flow electrochemical and flow photochemical processes are well established, there are virtually no reports on flow photoelectrochemistry - despite clear advantages! Developments in this area are thus urgently needed.
In this project, we will design, build and optimise a continuous flow set-up for photoelectrochemical reactions. The new setup will be used to develop energy-efficient synthetic methodologies for the preparation of industrially-relevant chemicals. We are particularly interested in photoelectrochemical C-C bond formation which is critical for the synthesis of advanced materials, pharmaceuticals and agrochemicals. The project will strongly benefit from the combined expertise of the supervisors in the areas of organic and radical chemistry, semiconductor synthesis and characterisation, photo- and electrochemical synthesis, chemical engineering, 3D printing and design and manufacture of complex devices. We anticipate that the new methodology will offer significant benefits for the applied organic synthesis compared to conventional approaches, and will in due course attract industrial interest.
The project is highly interdisciplinary and provides training in a wide range of device design and manufacturing, synthetic organic and inorganic methods, photo- and electrochemistry, and data analysis.
If you have any enquiries, please email Professor Victor Chechik
School of Physics, Engineering and Technology
Dr Mohammad Nasr Esfahani, Dr Richard Douthwaite, Prof Vlado Lazarov
To achieve the target of net zero emissions, both sustainable materials and advanced energy-related technologies will play important roles. In this context, hydrogen energy is considered one of the most promising for enabling global decarbonization and a clean energy transition. However, the storage, transportation, and processing of hydrogen are critical challenges to develop large-scale use. This is related to the diffusion of hydrogen in materials followed by degradation of properties and premature failure, known as “Hydrogen Embrittlement”, which has a direct impact on the energy price due to downtime and maintenance, or additional costs associated with possible passivation treatments. Hence, a fundamental and predictive physical mechanism is required to reveal the interaction of hydrogen with materials to inform the design of hydrogen-related facilities.
This project aims to provide a predictive mechanism for hydrogen embrittlement in metal crystals – mainly iron as a well-known sustainable material – through addressing the fundamental questions at the atomic scale using a combination of hydrogen diffusion experiments, state-of-the-art environmental transmission electron microscopy, and modelling. Experimental observations will be fed into numerical models to predict the hydrogen mobility in metal crystals and its implications on materials properties. We will collaborate with our commercial partners, JCB, who are a leading company in the use of sustainable machinery, to apply the predictive mechanism toward Hydrogen Internal Combustion Engines (H2-ICEs).
In this project, the student will receive a novel set of training skills on materials characterizations i.e. electron microscopy, mechanical testing, hydrogen- related failure analysis and Raman spectroscopy to test materials in hydrogen-related facilities. These skills will equip the student with knowledge and skills to predict potential hydrogen embrittlement phenomena in materials as well as addressing mitigation plans to improve sustainability. This project will engage with energy-related industrial partners to educate CDT with existing challenges and barriers to improve hydrogen-related technologies.
If you have any enquiries, please email Dr Mohammad Nasr Esfahani
School of Physics, Engineering and Technology
Professor Matt Probert, Dr Stuart Cavill
Background: Thermoelectric materials (TE) can improve energy efficiency and reduce dependency on fossil fuels, as they convert waste heat into useful electricity using a solid material with no moving parts. The Seebeck effect can operate whenever there is a temperature gradient: the greater the gradient, the more useful power that can be generated. The key challenge is to find a material that can generate a useful amount of power from modest heat sources, without using expensive or toxic elements.
Probert and his group have in recent years developed a complete workflow to predict zT, the key efficiency parameter, for any material from first principles using the CASTEP code. However, the whole process is slow, taking many months to characterise a single material. Ideally, we would have an efficient high throughput method that would enable many potential materials to be evaluated and hence accelerate discovery.
Objectives: The challenge for this PhD is to employ recently developed machine learning (ML) techniques within CASTEP to dramatically accelerate the key bottleneck parts of the calculation. Recent ML developments have accelerated CASTEP molecular dynamics (MD) by 10x. The student will replace the current use of the Boltzmann Transport Equation by MD- based techniques. This is a big change, and so they will first predict zT for some available materials that are not yet known to be thermoelectric, and then test the predictions by using the recently acquired PPMS (Physical Property Measurement System) to measure zT on real samples. Once the ML-accelerated methodology is validated, we can proceed to high-throughput screening to find better TE materials.
Training: The student will be trained by Probert in a variety of first principles simulation techniques and to code to a high standard, and by Cavill in the experimental measurement of transport properties.
If you have any enquiries, please email Professor Matt Probert
Department of Chemistry
Dr John Slattery, Dr Alison Parkin, Dr Phil Hasnip, Professor Matt Probert
The climate crisis requires us to transform the way that we generate, store and use energy. This joint experiment-theory project focuses on developing prototype devices and deepening our understanding of novel electrolytes, to enhance performance and sustainability in two key applications: longer-term grid energy storage and shorter-term energy storage, e.g. for electric vehicles.
We recently developed novel redox-active (“RA”) organic salts derived from ionic liquids (ILs), [RA][X], where [RA] + are a range of functional cations that can be oxidised and reduced to store/recover energy, and [X] - are anions that allow physical properties, solubilities etc. to be modified. [RA] + ions are based on naturally occurring building blocks, contain only earth-abundant elements, are non-toxic, simple to prepare and readily tunable. Their excellent solubilities, coupled with their favourable redox potentials, will enable cheaper, sustainable high energy-density storage which will out-perform current technologies. This has the potential to transform both redox-flow batteries (RFBs; for grid storage) and hybrid supercapacitors (HSs) (for electric vehicles). However, prototype devices need preparing, testing, and a fundamental understanding of operando ion-ion and ion-electrode electron-transfer processes is needed to optimise their performance.
This project combines synthetic work, advanced electrochemistry, device prototyping and state-of-the-art computational modelling to: 1) Expand and optimise our library of redox-active salts. 2) Prepare prototype RFBs and HSs from [RA] + salts and measure electrolyte long-term stability. 3) Perform electrochemical studies, including AC voltammetry and spectroelectrochemistry, to understand electron-transfer processes at the electrodes and link to electrolyte stability in devices. 4) Use high-performance computing and quantum-chemical models to understand organisation and reactivity of ions at the electrode/electrolyte interface and probe electron-transfer processes, again to understand and then optimise electrolyte performance and stability. The synergy between theory and experiment will allow unprecedented understanding of these salts. This will identify structural modifications to explore in the lab to improve performance, forming a feedback loop enabling rapid optimisation.
If you have any enquiries, please email Dr John Slattery