The Secrets of Solid-State Molecular Catalysis with Hyperpolarized Hydrogen
Collaborative work between researchers in York has demonstrated a new way to probe the catalytic cycle of molecular heterogeneous catalysts, by combining two areas of expertise in the Chemistry Department: advanced nuclear magnetic resonance (NMR) methods and new methods for the synthesis and reactivity of organometallic complexes in the crystalline phase.
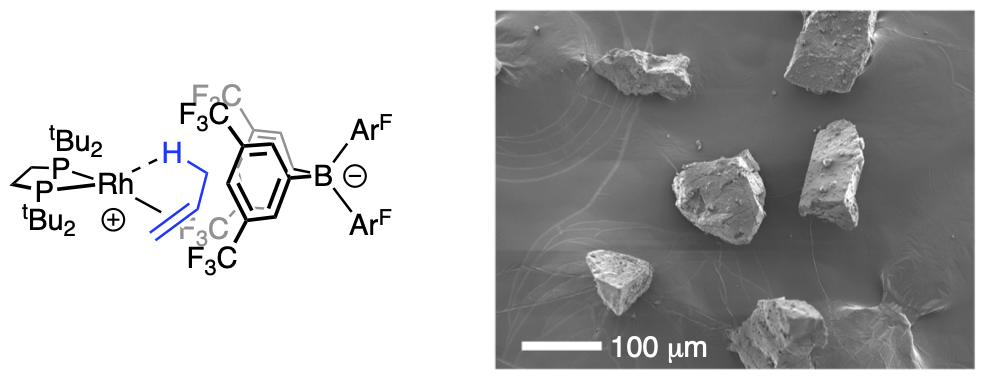
Catalytic processes are often conveniently divided into homogeneous or heterogeneous, and while both are important, industrial catalysis often operates using solid-state heterogeneous catalysts due to the benefits associated with catalyst stability, the physical separation of catalyst and substrates/products, operation in flow, and recyclability. Central to optimizing both types of catalysis, though, is the ability to define and control the catalytically active site(s); both to optimise the desirable properties of the catalyst (selectivity, rate) and also to stop decomposition of the catalyst. Defining the active catalyst sites in heterogeneous systems is very challenging.
Solid state Molecular OrganoMetallic (SMOM) chemistry is where molecular organometallic complexes are synthesised and undergo reactivity all in the solid-state, often as single-crystals. This is very different from normal organometallic chemistry where complexes are synthesised and studied in solution. SMOM catalysts offer several benefits of heterogeneous catalysis, such as higher catalyst stability and superior product separation. In addition, their well-defined molecular structures mean it is also possible to gain detailed insight into mechanism and catalyst speciation, that is usually only available for homogeneous catalysts in solution. This provides the opportunity to precisely tune catalyst structure and thus enhance reactivity or resistance to decomposition.
By combining SMOM chemistry (Weller) with expertise in advanced NMR methods that are usually used to analyse solution-based catalysis (Duckett), gas-phase NMR spectroscopy was used to measure the reaction of alkenes and alkynes with hydrogen using a crystalline rhodium-centred catalyst. By using parahydrogen, it is possible to achieve gas-phase NMR signals of the products with enhanced intensity as a result of the parahydrogen-induced polarization effect (PHIP). These enhancements are remarkable, with very high polarisation levels achieved (>85%) that results in signals that are almost 2000 times stronger than normal. This also results in gas phase 13C{1H} NMR spectra of the products of catalysis able to be analysed in a single scan in less than 1 second, an experiment that would normally take many hours to complete.
Gas-phase NMR spectrum of butyne hydrogenation that shows intermediate and final products in a single-scan gas phase 1H NMR spectrum at 25ºC.
Typically, PHIP requires the use of ~100% enriched parahydrogen, but with this catalyst, polarization transfer from the hydrogen to the products is so strong that, when coupled with fast catalytic turnover, the effect could be observed even using hydrogen taken directly from a standard cylinder, at the natural abundance of parahydrogen under standard conditions, resulted in significant enhancements.
Dr Matthew Gyton the first author on the paper and a PDRA in both Weller and Duckett groups said: “This study has opened up a simple way of achieving high polarisation levels in hydrocarbon products using solid-state organometallic catalysts. As well as being of fundamental importance in the field of catalysis, this also hints at future use of such catalysts in scenarios where hyperpolarised products are required for applications such as medical diagnostics.”
This research was as highlighted as an Editor’s Choice paper in the Journal of the American Chemical Society and was funded by the Leverhulme Trust and the EPSRC Catalysis Hub.